宇宙の幼少期を振り返り、最初の星々が瞬くのを目撃するには、まず家と同じ大きさの鏡を削る必要があります。その表面は、鏡が大陸のスケールである場合、足首の高さよりも大きな丘や谷がないほど滑らかでなければなりません。非常に巨大で滑らかな鏡だけが、空の最も遠い銀河から来るかすかな光を集めて焦点を合わせることができます。光は、ずっと前にその源を離れたため、宇宙が若かった太古の過去に現れた銀河を示しています。暗黒の中で神秘的な力が共謀し、星の最初の作物が輝き始めたとき、私たちが見る最もかすかな、最も遠い銀河はまだ誕生の過程にあります.
しかし、宇宙の歴史の初期の章を読むことは、最初の、おそらく巨大な星の性質を学ぶこと、重力によって星が形成される原因となった目に見えない物質、磁気と乱気流の役割、そして巨大なブラック ホールがどのように形成されるかについて学ぶことです。成長し、銀河の中心へと進みました — 並外れた鏡だけでは十分ではありません.
銀河形成の時代を誰も見たことのない理由は、太古の星の光が、何十億年にもわたって膨張する宇宙構造を通り抜けて私たちのところにやってきた後、引き伸ばされたからです。空の最も遠い星から放出された紫外線と可視光線は、ここまでの旅の間に約 20 倍長い波長に伸び、赤外線になりました。しかし、赤外線は、私たちが熱と呼んでいる原子の揺らめく光の一種であり、私たちの体や大気、足元の地面から放射されるのと同じ熱です。悲しいことに、これらの局所的な熱源は原始の星々の哀れな炎を飲み込んでしまいます。これらの星を観察するには、大きな完全な鏡を備えた望遠鏡を非常に低温にする必要があります。宇宙に打ち上げなければなりません。
問題は、家のサイズのミラーが大きすぎてロケットのフェアリングに収まらないことです。その場合、ミラーは折りたたむことができなければなりません。ミラーはセグメント化されている場合にのみ折りたたむことができます — 単一の途切れのない表面ではなく、ミラーセグメントのハニカム配列である場合.しかし、集合的に鮮明な画像を作成するには、空間内で自律的に展開した後、ミラー セグメントがほぼ完全に整列している必要があります。適切な焦点を合わせるには、驚くほど正確なモーターが必要です。モーターは、各ミラー セグメントをウイルスの幅の半分の増分で、すべて配置されるまで微調整できます。
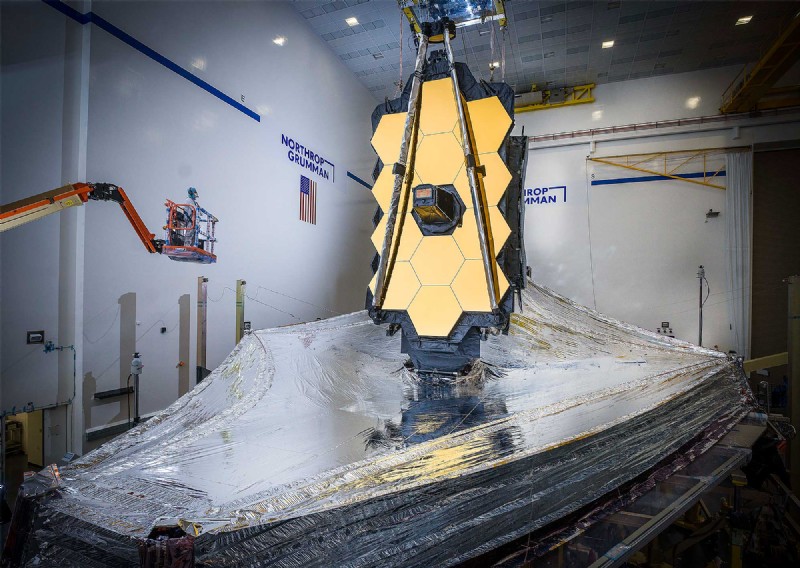
かすかな赤外線源を見る能力は、宇宙の形成期 — ビッグバンからおよそ 5000 万年から 5 億年後の期間 — にアクセスできるようにするだけでなく、宇宙の他の重要な側面も明らかにするでしょう。 、他の星を周回する地球サイズの惑星の特性から、宇宙が膨張している非常に争われている速度まで。しかし、望遠鏡が機能するには、空に撃たれた後に自律的に展開して焦点を合わせる完璧な鏡以外に、もう 1 つの要素が必要です。
宇宙空間でさえ、地球、月、太陽のすべてが依然として望遠鏡を加熱しすぎて、宇宙の最も遠い構造の薄暗いきらめきを認識できません.ただし、望遠鏡が、ラグランジュ ポイント 2 と呼ばれる月よりも地球から 4 倍離れた特定の地点に向かう場合を除きます。そこでは、月、地球、太陽がすべて同じ方向にあり、望遠鏡が 3 つの天体すべてを遮ることができます。テニスコートサイズの日よけを立てることで一度。このように日陰を作ると、望遠鏡はついに深い寒気に入り、ついに宇宙の夜明けの微熱を検出することができます。
日よけは赤外線望遠鏡の唯一の希望であり、アキレス腱でもあります。
ロケットを重くすることなく十分な大きさに展開するには、サンシールドは薄い生地で構成する必要があります。 (さらに言えば、鏡、カメラ、その他の機器、送信機、電源を含む天文台全体の質量は、地上に設置された大型望遠鏡の典型的な質量の約 2% にすぎません。)軽量の赤外線感知宇宙船を作るのは簡単ですが、生地の使用が避けられないため、本質的に危険な作業になります。技術者によると、ファブリックは「非決定論的」であり、その動きを完全に制御または予測することは不可能です。日よけが展開するときにひっかかると、望遠鏡全体が宇宙ゴミになります。
現在、信じられないほど組み立てられた望遠鏡は折りたたまれており、アリアン 5 ロケットの上に配置する準備ができています。ロケットは、ペイロードであるジェームズ ウェッブ宇宙望遠鏡 (JWST) が最初に構想されスケッチされてから 30 年以上経った 12 月 22 日にフランス領ギアナのクールーから打ち上げられる予定です。望遠鏡は予定より 14 年遅れており、予算を 20 倍上回っています。 NASA 主導のプロジェクトで 25 年間主任科学者を務め、ノーベル賞を受賞した天体物理学者であるジョン メイザーは、次のように述べています。今、彼は言った、「私たちは何十億ドルもの望遠鏡を爆発物の上に置き、物事を運命に変えます.
過去 30 年間にわたる JWST の開発の物語は、特に Webb の前任者のおかげで、宇宙の理解において私たちが成し遂げた途方もない進歩と並行してきました。ハッブル宇宙望遠鏡によって、恒星、銀河、超大質量ブラック ホールが、宇宙の歴史の中で誰もが予想していたよりもはるかに早く存在し、その後急激な変化を遂げていることがわかりました。私たちは暗黒物質と暗黒エネルギーが宇宙を形作ることを学びました。ケプラー望遠鏡などを使って、あらゆる種類の惑星がクリスマス ツリーのつまらないもののように銀河を飾っているのを見てきました。これらの発見は、ジェームズ ウェッブ宇宙望遠鏡が対処できる疑問を提起しました。天文学者はまた、他の望遠鏡と同様に、その目撃が新しい疑問を提起することを望んでいます. 「新しい機器を作るたびに、驚きがあります」と Mather 氏は言います。
打ち上げは、天文学者のナタリー・バターリャが「ピンと針の 6 か月」と呼んだものから始まります。これは、驚くほど複雑な望遠鏡が数百のステップで展開し、焦点を合わせようとするためです。この天文台は、ラグランジュ ポイント 2 まで 100 万マイルを 1 か月かけて浮遊します。その途中で天体のスイレンに変わり、金メッキされたミラー セグメントの巨大な花がさらに大きな銀箔の上に配置されます。
ハーバード大学の天体物理学者で、望遠鏡の時間割り当て委員会のメンバーだったグラント・トレンブレイ氏は、「それは私たち自身の『大胆な挑戦』の瞬間になるでしょう」と語った。 「それは驚くべきことをするでしょう。 The New York Times に掲載されます これがどのように時の果てに星の誕生を目撃しているかについて話しています。これは最も初期の銀河の 1 つであり、これは他の地球の物語です。」
「仕事をお願いします」トレンブレイは目を上に向けて付け加えた.
スムーズからゴツゴツへ
NASA がこれほど重要な天文台を最後に打ち上げたのは 1990 年のハッブル宇宙望遠鏡でしたが、それは大惨事でした。ベテランの天文学者、サンドラ・フェイバーは私にこう言いました。フェイバーは、メリーランド州グリーンベルトにある NASA のゴダード宇宙飛行センターでキャンプを行い、障害を診断するチームに所属していました。ハッブルの写真の星がリングのように見える様子から、彼女と同僚は、主鏡 (光を副鏡に反射させ、カメラのレンズに反射させる大きな凹面鏡) が研磨されていないことを推測しました。光を集中させるのに最適な凹面まで下げます。エッジの周りでは半波長分厚すぎました。主鏡と副鏡が打ち上げ前に一緒にテストされていれば、この異常に気づいていたでしょうが、大幅に遅れて予算をオーバーした望遠鏡を打ち上げようと急いでいたため、そのようなテストは行われませんでした。
一部の NASA リーダーは、すでに物議を醸しているプロジェクトであった望遠鏡を放棄するよう求めました。代わりに、メリーランド州のバーバラ・ミクルスキー上院議員が救助活動のための資金を確保しました。ハッブルは、赤外線ではなく虹の色に敏感な光学望遠鏡として、わずか 340 マイル上空にある地球低軌道から、100 万マイルも移動する必要がなく、鮮明な視界を得ることができるため、修正が可能でした。 . 1993 年、スペースシャトルがハッブル宇宙望遠鏡とドッキングし、宇宙飛行士が一種のコンタクト レンズを装着しました。この望遠鏡は、天文学と宇宙論に革命をもたらしました。

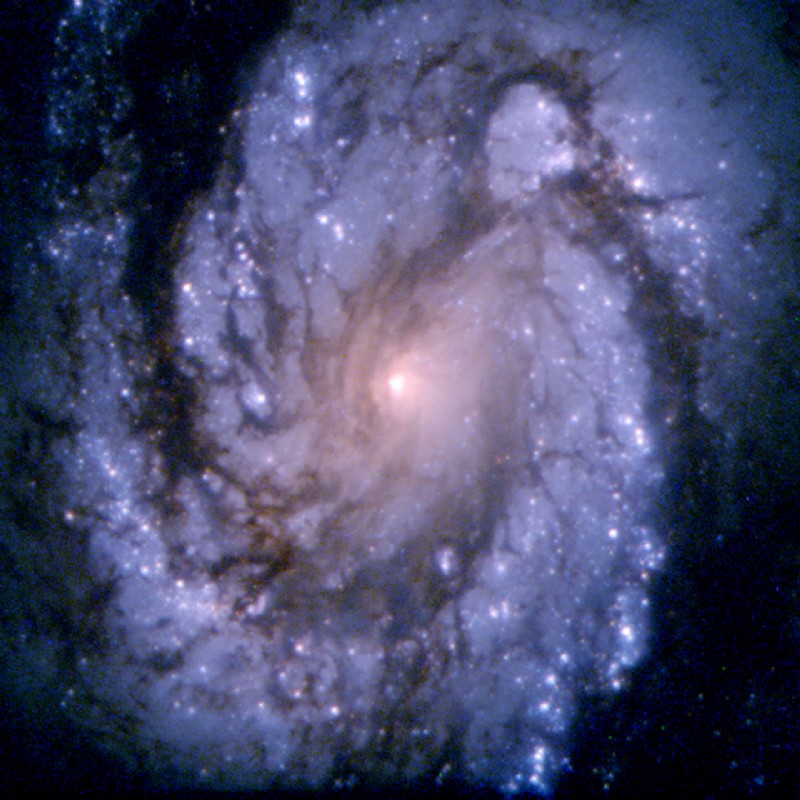
おそらく、20 世紀の大部分において、宇宙に関する最も重要な問題は、宇宙に始まりがあったのか、それともずっとそうであったのかということでした。英国の宇宙学者フレッド・ホイルと後者の「定常状態」理論を信じる他の人々にとって、「説得力のある論理はシンプルでした」と、天文学者でウィスコンシン大学マディソン校の名誉教授であるジェイ・ギャラガーは言いました。 「ある時点で何かが変化し、宇宙が物質を作り出したのはなぜですか?」定常状態の支持者であるホイルは、彼のライバルの「ビッグバン」(彼はそれを吹き替えた) に対する信念は創世記の影響によるものだと考えた。
その後、1964 年にニュージャージー州のベル研究所のラジオ アンテナでヒスノイズが発生しました。ヒスノイズは、ビッグバン理論で予測されたとおり、空のあらゆる場所から到達するマイクロ波によって生成されました。 (この光は、熱く高密度の宇宙が冷却する初期の相転移で放出されました。) 宇宙マイクロ波背景放射と呼ばれる現象が発見されても、すぐに議論が終結することはありませんでした。さらに何十年もの間、彼らの理論に。しかし、ビッグバンを見たときにその残光を認識した他の人にとって、CMB はパズルを作成しました。空のすべての部分から来るマイクロ波のほぼ完全な均一性は、生まれたばかりの宇宙が驚くほど滑らかで、物質のピューレであることを示していました。 1960 年代後半に銀河を研究していた大学院生だったフェイバー氏は、「謎は、今日、非常にゴツゴツした宇宙が見られることです」と述べています。 「したがって、銀河を理解する上での最初の課題は、宇宙がどのように滑らかな状態からでこぼこの状態になるかを理解することです。」
宇宙学者は、原子が重力のために徐々に凝集し、最終的に星や銀河のような構造に分裂したに違いないことを知っていました。しかし、紙の上では、構造の成長は非常に遅かったようです。物質は最初は滑らかに分布し、重力によって特定の方向に引っ張られなかっただけでなく、空間の膨張と光自体によって生成された圧力の両方が物質を分離するように働き、その弱い重力を打ち消しました.

暗黒物質に入ります。 1970 年代に、ワシントンのカーネギー研究所のベラ ルービンは、銀河の周辺が予想よりもはるかに速く回転していることを観察しました。暗黒物質と呼ばれる銀河内およびその周辺に実質的に存在しない物質があるというこの証拠は、1930 年代にフリッツ ツヴィッキーが観察した、銀河はその発光物質のみに基づく必要以上に互いに引き付け合うように見えるという観察と一致しました。また、1970 年代に、プリンストン大学のジム・ピーブルズとジェリー・オストライカーは、星、ガス、塵だけで構成される回転する銀河円盤が不安定になり、球状に膨らむはずだと計算しました。彼らは、目に見えない物質が、目に見える円盤が回転するより強力な重力井戸を作り出しているに違いないと仮定しました。 1979 年、フェイバーとギャラガーは、暗黒物質のすべての証拠をまとめた有力な論文を書き、宇宙の物質の約 90% を突き止めました。 (現在の見積もりは約 85% です。)
これらの研究者は、かなりの重力と光の圧力に対する不浸透性を備えた暗黒物質が、初期の宇宙で比較的急速に集まった可能性があることに気付きました。宇宙論への貢献により 2019 年のノーベル物理学賞の半分を受賞したピーブルズは、暗黒物質の粒子がまとまって塊 (ハローとして知られる) になり、それが結合してますます大きな塊になるという定性的な図を作成しました。英国の天体物理学者であるサイモン ホワイトは、原始的な 1980 年代のコンピューター シミュレーションで、この「階層的クラスタリング」プロセスを実証しました。当時、目に見える物質は複雑すぎてシミュレートできませんでしたが、研究者たちは、暗黒物質の集塊が光る物質を運んだのではないかと推測しました。暗黒物質の輪の中に閉じ込められた原子は、互いに衝突し、加熱され、中心に向かって沈み、最終的には沈みました。星や円盤状の銀河に重力崩壊します。
ほとんどの宇宙論者はこのイメージに納得しましたが、大きな問題は、物質の密度の変化が最初にどのように始まり、重力クラスター化プロセスを急上昇させたかということでした。 「人々は、宇宙構造の形成に関する合理的な初期条件が何であるかについて明確な考えを持っていませんでした」と、現在引退してドイツに住んでいるホワイトはズームで私に語った。 「これらのシミュレーションを実行することはできましたが、最初は何を入れればよいかわかりませんでした。」
「SPECTACULAR REALIZATION」、宇宙学者のアラン・グースは 1979 年にノートに走り書きしました。彼は、ビッグバンの開始時に空間が気球の表面のように突然爆発したとすれば、それがいかに巨大で滑らかで滑らかな空間になったかを説明できると計算していました。平らな。 Guth が原初の成長スパートと呼んだ宇宙のインフレーションは、ビッグバンのアドオンとして急速に人気を博しました。宇宙学者はすぐに、インフレーションの間、空間が爆発するにつれて空間構造の量子変動が凍結し、宇宙全体に微妙な密度の変化が生じたことに気づきました。インフレによって作成された推定密集スポットは、将来の構造の種として機能した可能性があります。
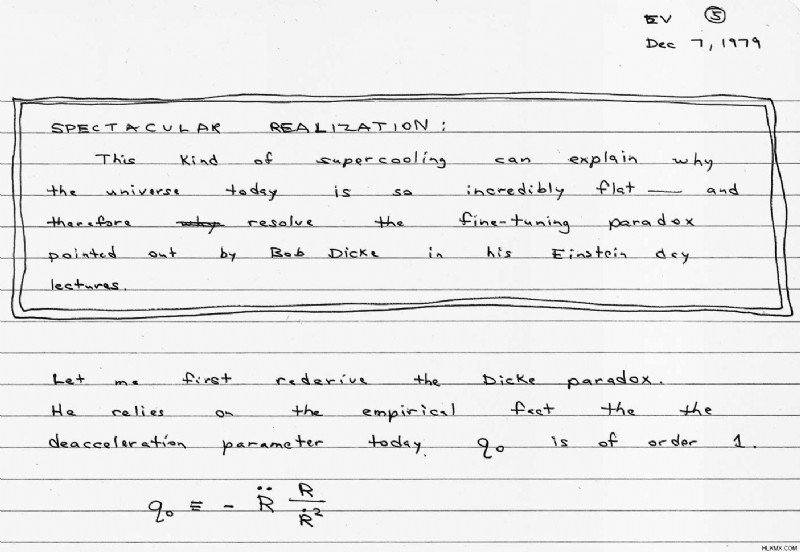
これらの小さな密度の変化は、1990 年代初頭に CMB で実際に測定されました。この偉業により、Webb 望遠鏡のトップ科学者であり、ノーベル賞を受賞しました。しかし、それらが測定される前でさえ、フェイバーのような人々は密集したスポットをプロットに取り入れていました. 1984 年、彼女と 3 人の共著者は Nature に論文を発表しました。 それがすべてをつなぎ合わせました。 「これは、インフレーションがどのように変動を引き起こし、変動が後に銀河を作るために何をするかについての最初の簡単な説明です」と彼女は言いました.
しかし、話は最初から最後まで憶測でした。そして、それが大まかに真実であったとしても、重要な日付と詳細は不明でした.
ハッブル望遠鏡の最も影響力のある発見の 1 つであり、後継の Webb を構築する大きな原動力となったのは、補正レンズが設置されてから 2 年後の 1995 年でした。当時ボルチモアの宇宙望遠鏡科学研究所所長であったボブ・ウィリアムズは、ウェッブと同じようにハッブルのオペレーションセンターであり、何人かのポスドクの提案で、彼の「所長の自由時間」の 100 時間すべてを捧げることに決めた。ハッブルを望むところならどこにでも向けることができたし、何にも向けることができなかった - サムネイルの月よりも狭く、暗くて特徴のない空の小さなパッチ。アイデアは、感度の低い望遠鏡の手の届かないところに隠れていた可能性のある、信じられないほどかすかな遠くの物体を探すことでした。
同僚はこれはもったいないと考えました。故ジョン・バーコールは、ウィリアムズを説得しようとした。有名な天体物理学者である Bahcall と彼の妻である Neta Bahcall は、星や銀河のような構造が宇宙史の比較的後期に出現したという典型的な考えを持っていました。もしそうなら、遠い遠い昔のかすかな天体を解決しようとしてもうまくいきません。 Bahcalls と他の多くの理論家は、ウィリアムズの写真は暗いものになるだろうと考えていました。
しかし、100 時間の暴露の間に、宝箱の蓋が開いた。小さな長方形の空間が、あらゆる形、大きさ、色合いの何千もの銀河で輝いていた。天文学者は唖然としました。

ハッブル ディープ フィールドの写真で遠くにある銀河は、より赤く見えます。これは、それらの光が膨張する空間をより長く移動してここに到達したため、より長い波長に引き伸ばされた、つまり「赤方偏移」したためです。この色分けにより、ディープ フィールド画像は宇宙の 3D ビューと銀河の進化のタイムラインを提供します。銀河はすべての年齢と発達段階で現れます。これは、宇宙が時間の経過とともに根本的に変化したことを証明しています。 「窓の外に出て、二度と聞かれなくなったのは、定常状態の理論でした」とフェイバーは言いました。 「望遠鏡で1枚の写真を撮ることができ、過去を振り返ることができ、当時の宇宙が別の獣だったことを知ることができたのは、大きな知的ブレークスルーでした。」
写真は、ほとんどの専門家が予想していたよりもはるかに速く宇宙で明るい天体が形成されたことを示しました。これは、それらが重力の強さだけで形成されたのではなく、合体する暗黒物質のハローの背中に乗って運ばれたという理論を補強しました.
初期の銀河は奇妙に見えました — 白鳥に成長するのに何十億年もかかる醜いアヒルの子のように、小さくて乱れていました。 「今日の美しい[渦巻銀河と楕円銀河]を含む美しい宇宙は、本当に後期開発のようなものです」とフェーバー氏は言いました。アヒルの子銀河のいくつかは衝突して合体しており、宇宙構造の成長に関する階層的クラスタリング理論を支持しています。また、太古の昔の銀河の星の塊は驚くほど明るく、現在の太陽型の星よりもはるかに質量が大きく明るいことを示しています。
天文学者は、ほとんどの銀河がピーク光度に達し、最も急速に星を形成することを観察しました。これは、「赤方偏移 2」の付近です。これは、光がここに到達するまでに、発光波長の 2 倍に伸びた距離であり、ビッグバンから約 20 億年後に相当します。その後、銀河の中心で成長している神秘的な超大質量ブラック ホールに関連していると現在考えられている理由により、多くの銀河が暗くなりました。
しかし、ディープ フィールドの写真に見られる銀河の進化のタイムラインで最も印象的なことは、始まりが見えないことです。ハッブルのガラスの目が見える限り、銀河がありました。宇宙飛行士が後に望遠鏡に取り付けたアップグレードされたカメラで撮影されたさらに深い視野の写真では、ビッグバンから約 5 億年後に相当する赤方偏移 10 まで離れた光のしみが一時的に発見されました。現在では、その何億年も前に構造が形成され始めた可能性が高いと考えられています.
しかし、形成過程にある銀河は、その物質が初めて星に分裂し、ハッブルが検出するには遠すぎてかすかであり、赤方偏移も大きすぎます。これらの銀河からの光は、銀河の目に見える部分からまっすぐ伸びています。電磁スペクトルと赤外線に。それらを見るには、より大きな赤外線感知望遠鏡が必要です。
「ハッブルがハッブル・ディープ・フィールドで成功したことは、私たちが考えていたよりもはるかに高い赤方偏移に銀河があったことを発見したことです」とネタ・バーコールは私に語った。 「James Webb への質問は、それがいつ始まったのか、そしてどのようにしてそんなに早く始まったのかということです。」
ワズーの外に出る惑星
1995 年 10 月、ハッブル宇宙望遠鏡が何も見つめずに時間の歴史を垣間見る 2 か月前に、スイスの天文学者ミシェル マヨールは、イタリアのフィレンツェで開催された会議で別の主要な発見を発表しました。スター。
市長の講演会の講堂の後ろで、当時カリフォルニア出身の大学院生だったナタリー・バタリャは、今聞いたことの重要性を認識できませんでした。 「これらのことがどのように起こるかは面白いです。振り返ってみると、それは極めて重要な瞬間だったからです」とバターリャは最近、彼女の仮想背景で星を周回する 3 つの惑星に囲まれて語った。 「それは太陽系外惑星探査のこの新しい時代の幕開けでしたが、私の人生の変革の瞬間でもありました。私はまだそれを知りませんでした.」

当時、太陽系外惑星探索は科学的な後進国であり、メイヤーとケロースの方法は見込みのないものに思えました。星の光を色成分に分割するスペクトログラフを使用して、100 以上の太陽のような星を監視し、ドップラー シフトを検出しようとしました。これは、軌道を回る惑星の重力によって乱されているため、星が揺れていることを示している可能性があります。利用可能な最高の分光器で見られるように星をぐらぐらさせるには、惑星は馬鹿げたほど重く、主星の近くになければならないため、この手法はとてつもないものに思えました。しかし、メイヤーとケロースが 50 光年離れた太陽のような恒星であるペガスス座 51 を見たとき、ぐらつきは大きかった.太陽から水星までの距離。
メイヤーとケロースは太陽系外惑星を手に入れただけでなく (最終的には、2019 年のノーベル物理学賞の残りの半分をピーブルズと共有しました)、惑星自体、ペガスス座 51 番星 b は、太陽系とは何かについての教科書の理解を独力でひっくり返しました。お気に入り。惑星科学者のハイディ・ハンメルが言ったように、「私たちは太陽系がどのように形成されたかについての素敵なおとぎ話を教えられました」。このおとぎ話は、岩石惑星が星の近くにあり、巨大なガスと氷の惑星が遠くに形成される理由を説明するために設計されました.では、「熱い木星」であるペガスス座 51 番星 b は実際に太陽をかすめながら何をしていたのでしょうか?
バターリャは、市長のプレゼンテーションに対するフィレンツェの聴衆の反応を覚えています — 沈黙.しかし、すぐに、懐疑論はより多くのホットジュピターの発見に取って代わられました。そして、望遠鏡と技術が向上するにつれて、他の太陽系外惑星も現れました.フィレンツェでのその日から 16 年後、バターリャは NASA チームを率いて、確認された最初の岩石系外惑星、ケプラー 10b を発見しました。

カリフォルニア州のイーストベイで育ったバターリャ (当時はナタリー スタウト) は、科学についてほとんど考えたことがありませんでしたが、17 歳のとき、1983 年のサリー ライドの宇宙旅行に興奮しました。カリフォルニア大学バークレー校、ビジネス専攻として入学。しかしその後、2 年生のある週末、洗濯をしていると、突然自分にこう言ったのを覚えています。この世界で何かできるとしたら、それは何だろう?」そして驚くべきことに、すぐに答えが返ってきました。それは、宇宙プログラムで働くことでした。
彼女は物理学のクラスに入学しました。彼女は苦労しましたが、それを愛していました。毎日の出来事が数式で説明できることは、「私の人生に意味を与えてくれました」とバターリャは言いました。 「それは、宇宙における自分の場所を違った見方をさせてくれました。油の水たまりが虹色のきらめきを作る理由である、薄膜からの干渉を説明する方程式を書けるとしたら、「自然界について私たちが知ることができることには、どのような限界があるのでしょうか?」
Batalha は、何らかの方法で科学とビジネスを組み合わせたいと考えていました。彼女は授業を受け続け、数少ない地上の赤外線望遠鏡の 1 つであるワイオミング赤外線天文台で夏のインターンシップに参加しました。彼女がそこに着いたとき、彼女はアドバイザーとコホートに、自分は彼らのようではなく、科学者になるつもりはないと言いました.しかし、彼女の顧問が彼女に取り組まなければならない問題を与え、彼女がそれを解決し、それが後に出版につながったとき、彼は彼女に「自分を安売りしないでください」と言い、彼女がバークレーに戻ったらジボール・バスリのドアをノックするように彼女は言った. .
星の天文学者であるバスリは、星のスペクトルを分析する仕事を彼女に課しました。科学的な方法を直接体験したことで、彼女は夢中になりました。彼女はまた、オフィスメイトでバスリのポスドクであるセルソ・バターリャにも恋をした。彼女は彼と結婚し、カリフォルニア大学サンタクルーズ校の天文学の大学院に進み、息子のノーランと娘のナターシャを立て続けにもうけました。さらに2人の子供が続きます。その間、彼女とブラジル人のセルソ・バターリャは、カリフォルニアとリオデジャネイロの間を家族で行き来しました。リオでの生活は美しいものでしたが、複雑でした。彼女はスラム街を車で通り過ぎ、道路脇に黒焦げの死体を見たのを覚えています。彼らは最終的にベイエリアに定住しました。
バターリャは、主に天文学を行う母親であると考えており、会議には一度も出席しませんでした。しかし、1995 年の秋にウィーンで開催された星の分光会議で、星のスポットに関する新しいデータを発表するよう招待されたとき、彼女は例外として、両親をヨーロッパに連れて行き、末っ子の子守をしました。彼女は、翌週フィレンツェで開催された別の惑星に関する会議に出席することにしました。バターリャが、土壇場でスケジュールに追加された講演のためにテレビカメラがセットアップされていることに気付いたのは、2 回目の会議の最終日でした。 「その後、ミシェル・マイヨールが起き上がり、この惑星の発見についてさりげなく話します」と彼女は言いました。
最初、バターリャは新しく発見されたホットジュピターについてほとんど考えず、サンタクルスの星のスポットを研究し続けました.それから 1 年かそこら後、シリコン バレーにある NASA のエイムズ研究センターの科学者、ビル ボルツキという名前の科学者の話を聞きました。彼は巨大ガス惑星だけでなく、地球サイズの岩石系太陽系外惑星を検出できる宇宙望遠鏡を作ることを決意していました。 Borucki はトランジット法を使用することを計画しました:Mayor と Queloz が行ったように星明かりの色の変化を追跡する代わりに、彼は星の前を周回する惑星が横切ることによって引き起こされる星明かりの強度の周期的な落ち込みを探します。その光の。
バターリャはこれがうまくいくとは思わなかった。彼女がたまたま知っていた星の斑点は、地球とほぼ同じ大きさです。そのため、トランジットする小さな惑星は、回転する星の周りを一掃する星のスポットと見分けがつかないだろうと彼女は考えました。彼女はこの問題についてボルツキに手紙を書きました。彼は返事を書き、NASAはまさにその理由の一部で彼の提案を拒否したと言いました.彼女は彼と一緒にエイムズに来て、岩石の世界から星のスポットを区別する方法を見つけ出しますか?
彼女はそうするでしょう、そして彼らはそうしました。次回、NASA は Borucki の提案を承認し、Batalha はプロジェクト科学者になりました。ボルツキと彼のチームによって設計されたケプラー宇宙望遠鏡は、トランジットする惑星の沈下を探して約 150,000 個の星の明るさを継続的に監視するために設計されましたが、2009 年 3 月に打ち上げられました。 .

ケプラーは地球サイズの惑星で配信されます。 「ケプラー 10b は、探査機から取得した最初の 10 日間のデータで特定されました」とバターリャは言いました。彼らが主星の明るさを経時的にグラフ化すると、その落ち込みが目に見えました。地上からの追跡調査により、それが本物の惑星であり、その質量と半径に基づいて、岩石であることが確認されました。バターリャは、ヨーロッパの天文学者による CoRoT-7b とラベル付けされた岩石系外惑星のより暫定的な主張に続いて、2011 年 1 月に明確な検出を発表しました。ケプラー 10b も CoRoT-7b も、水が液体である「ハビタブル ゾーン」ではなく、親星の近くを周回していたため、切望されていた「地球のような」指定を獲得できませんでした。 (2014 年には、岩石が多く、水が多く、地球に似ている可能性がある最初の惑星、ケプラー 186f が注目を集めました。バターリャはこの分析に正式に関与していませんでした。)
ケプラー望遠鏡は、2 つのモーターの故障によって時期尚早に足止めされる前に、2,600 以上の太陽系外惑星を発見しました。全部で 4,500 個以上が数えられており、天文学者がそれらの統計的特性を研究するには十分な数です。ペガスス座51番星bが示唆したように、私たちの太陽系は非典型的です。たとえば、銀河で最も一般的なタイプの惑星は、岩石惑星と巨星の間の、私たちが持っていないサイズです。惑星天文学者は、これらのいわゆるスーパーアースまたはサブ海王星の余剰、またはこれらの中型惑星がどのようなものか、またはそれらがどのように形成されるかをまだ理解していません.惑星の形成と進化の新しい原則が必要です。
これまでのデータを推定すると、研究者は、私たちの銀河には何十億もの岩石と水の惑星があると考えており、生命も一般的である可能性があることを示唆しています.生命が実際に別の惑星に生息しているという証拠が見つかるまでは、生命が地球上に現れたのはまぐれであり、私たちだけである可能性はあります。
幸いなことに、Webb 望遠鏡は、他の地球の大気や気候を調査するのに十分強力です。運が良ければ、実際の異星人の生物圏の証拠を見つけることさえできます。
「赤外線は太陽系外惑星にとって素晴らしいものです」とバターリャは言いました。
一撃でアウト
1987 年のある朝、当時宇宙望遠鏡科学研究所 (STScI) の所長であり、まだ打ち上げられていなかったハッブルの所長であった天体物理学者のリッカルド ジャコーニは、副所長のガース イリングワースにハッブルの後継者について考え始めるように依頼しました。 「私の即座の反応は、『ああ、ハッブルはまだ打ち上げられていません。そこには何百万ものやるべきことがあります。それには大きな問題があります。それでは、どうすればこれを行うことができるでしょうか?』」と Illingworth 氏は回想します。近々。 「彼は言った、『信じてください、これを行うには何年もかかることを私は知っているので、あなたは早く始めなければなりません』.プリンストンのライマン・スピッツァーによるキャンペーン。彼らはハッブルの母と父として知られています。
オーストラリア出身の Illingworth 氏は、STScI の同僚であるフランスの Pierre Bely 氏と米国の Peter Stockman 氏と集まり、次世代の宇宙望遠鏡についてブレインストーミングを行いました。彼らは基本的に何もすることがありませんでした。 「私たちは、ハッブルを超えて、ハッブルが行ったことを補完し、新しい分野を探求するために何が良いかを考え始めました」とイリングワース氏は言いました。赤外線は地上から観測するのが非常に困難です。このトリオは、赤外線背景が 100 万分の 1 以上低い宇宙では、見るべきものがたくさんあるだろうと考えました。 「強力な新機能を導入すると、膨大な数の科学的地平が開かれます。」
IR 望遠鏡が、幅 2.4 メートルの主鏡を備えたハッブルのように高感度であるためには、より大きな波長を検出するため、Illingworth、Bely、および Stockman は、それを大幅に大きくする必要があることに気付きました。 They considered that the mirror might have to fold to fit in a rocket. They also knew it had to be cold, otherwise its heat would saturate its own sensors. Rather than actively cool the telescope, they thought to exploit the extreme frigidity of outer space by blocking the heat of the Earth, moon and sun. Their vague conception of a large, passively cooled infrared telescope, greatly elaborated upon, would become the cargo now awaiting launch in Kourou.
Leading astronomers convened at STScI in 1989 to discuss the science that an infrared space telescope might be good for. Discussions slowed during Hubble’s disastrous start and salvation, then picked up again in the mid-’90s. In 1995, John Mather, a reedy, gentlemanly astrophysicist at the Goddard Space Flight Center, got a call from NASA headquarters asking if he’d like to join the project. Realizing that an infrared telescope “would do so much for so many people,” he dropped everything and signed on. He’s been JWST’s top scientist ever since.
Mather calls himself a “theoretical instrument builder.” He started building telescopes as a kid in pastoral New Jersey, assembling parts from catalogs in the hope of getting a closer look at the surface of Mars. As a young man in the 1970s, Mather worked on a balloon-borne instrument that failed; he and his colleagues concluded that they hadn’t tested it enough before launch. “Murphy’s law had been proven one more time,” he wrote in an autobiographical account. But lessons learned led to the triumph of COBE, the NASA satellite experiment for which he and George Smoot would share the Nobel. In the early ’90s, COBE measured the subtle variations in the cosmic microwave background that are thought to have seeded all later cosmological structures. In Mather’s mind, theorizing about the cosmos is fine, but you need ingenious instruments to know anything for sure. “So let’s build the equipment,” he told me this fall. “To me that’s a heroic thing to do.”

Mather had contemplated wild designs, including telescopes that fold. In the difficult budgetary climate of 1996, however, a committee of top astronomers studying the infrared telescope concept proposed a 4-meter mirror, which would fit in a rocket fairing, dramatically cutting costs and complexity. Illingworth thought this was “stupid. It was not going to be as good as Hubble.” NASA’s leader at the time, Dan Goldin, evidently felt similarly. At the American Astronomical Society meeting that year, Goldin said in an address:“Why do you ask for such a modest thing? Why not go after 6 or 7 meters?” As committee member Wendy Freedman remembers it, “Goldin essentially said, ‘You guys are really a bunch of scaredy-cats.’” He got a standing ovation. “In my mind he saved the telescope,” said Illingworth. It would be bigger. It would also have to fold after all.
After some heady talk about 8 meters, in 2001 NASA finally settled on 6.5 meters for the segmented mirror’s diameter, giving the next-generation telescope more than six times Hubble’s light-collecting area. The question was:How can you fit a 6.5-meter-wide mirror in a 5.4-meter-wide rocket fairing?
“A big part of the design is how do you fold it up,” Mather said. Outside contractors developed competing mirror designs. Lockheed Martin’s mirror folded like six petals of a flower, Ball Aerospace’s like a drop-leaf tabletop. TRW proposed putting mirror segments in place the way an old jukebox puts records down. After mulling over the proposals for a year, Mather and his team adopted bits and pieces from each. The main contract went to TRW because of the company’s extensive experience building complex satellites for the U.S. military and its successful construction of the Chandra X-ray observatory. (TRW was soon purchased by Northrop Grumman.) The mirror design would be closer to Ball Aerospace’s:an array of 18 hexagonal segments forming a larger hexagon that would fold on two sides. Mike Menzel, who spearheaded Lockheed Martin’s proposal, was brought on by NASA as Webb’s chief engineer.
The mirrors would be made of beryllium — light, strong, stiff stuff that’s toxic in powder form (“Beryllium is a pain in the neck, but it’s the only thing that would work,” according to Mather). Powdered beryllium was pressed into blocks in Ohio, then cut to shape in Alabama. The 18 mirror segments were then topped with a layer of gold, which is supremely reflective of infrared light, and polished in a California factory built specifically for the purpose. “Shaping and polishing telescope mirrors is a dark art that goes back hundreds of years,” said Sarah Kendrew, a Belgian-British astronomer who works on MIRI, one of Webb’s instruments.
Motors with unprecedented finesse would be needed to bring the hexagonal mirror segments into collective focus in space. “That’s something we had to invent right away,” Mather said. “If you can’t do that, you can’t make the whole observatory work.” Ball Aerospace delivered actuators capable of nudging each of the gold hexagons in 10-nanometer increments, one ten-thousandth the width of a hair. Mather said the motors work by “flexing,” or “converting a big motion into a tiny motion,” though Ball’s design, despite being taxpayer-funded, is proprietary. “When we take a picture of the telescope we have to make sure no one could see the motors,” he said.

In 2002, the telescope got a name. NASA administrator Sean O’Keefe broke a tradition of naming telescopes for scientists — the Hubble telescope, for instance, refers to the American astronomer Edwin Hubble — and instead honored an earlier administrator, James Webb, who was head of the space agency during the Apollo era. The choice was immediately unpopular with astronomers and has grown increasingly so. Last year, 1,200 astronomers signed a petition to rename the telescope after claims that Webb either aided or chose to ignore the firing of gay government employees during the Lavender Scare. After an investigation, NASA announced in October that historians found no evidence warranting a name change.
Various institutions, from the University of Arizona to the European Space Agency, signed up to build the cameras, spectrographs and coronagraphs that will swivel into place at the focal point of the optics, slicing and dicing different chunks of the pooled infrared light. In exchange, these institutional partners will command extensive telescope time.
As for the sunshield, the flimsy material on which the infrared telescope’s fate rests, the team quickly settled on Kapton, a slithery silver plastic that looks like the inside of a potato chip bag but has the thickness of a human hair. Since it might tear, the sunshield would need many layers for redundancy — the team decided on five — and the layers would have to be unfurled, separated and held taut by a system of booms, cables and strings. Propulsion systems and solar panels would go on the sunward side, and the optics and instruments, which must operate below minus 223 degrees Celsius, would huddle on the dark side. “JWST has a lot of firsts, an awful lot of significant firsts,” Menzel, the chief engineer, told me, “but that sunshield is one of them.”
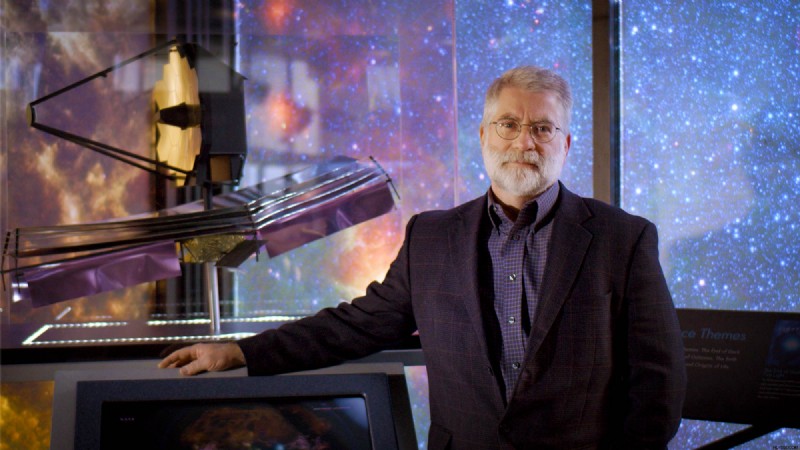
Menzel, who is thickset with a neatly clipped gray beard, oversees thousands of people’s work on one of the most complicated engineering projects in history; he’s also the type of person who tells you right away where they’re from. That would be Elizabeth, New Jersey — Exit 13 off the turnpike — where his father drove a cab. On a recent Zoom call, Menzel bent his arm back and forth at the elbow to explain the challenge presented by the sunshield. “If you take something rigid, like a door, and you build a nice hinge, you can predict the way that moves,” he said. “That’s a piece of cake.” He stopped bending his arm. “Now you got blankets. They’re floppy. Try to push on a blanket on your bed and predict the shape that it’s going to go in. It’s horrible. Same thing with a string — the strings that tension [the sunshield]. There’s a million different ways that a string can move.” It gets worse:“Now put all this experience in zero-G, where that stuff can go in places you just don’t want it to go.” Smoothly unfolding the sunshield “becomes a very tough problem.”
Around 2004, the NASA engineers Chuck Perrygo and Keith Parrish came into Menzel’s office at Goddard and said they had a way to do it. Perrygo picked up a piece of paper on Menzel’s desk and folded it into the shape of a Z. The sunshield could be folded into many more such zigzags, in what’s sometimes called an accordion fold. “I’m pretty good at recognizing a bad answer, and I’m pretty good at recognizing a right answer,” Menzel told me, holding up a piece of paper he had folded into a Z shape. “So we all saw that and thought, that’s a way to pursue it.” Northrop Grumman was separately coming to the same conclusion.
The next question was how to hold the accordion fold in place until the sunshield was ready to unfurl. A Northrop Grumman engineer, Andy Tao, found the solution:107 pins that retract like a cat’s claws.
The pin approach sprouted another tricky problem:pins make pinholes. If, after unfurling, pinholes on all five Kapton layers were to line up, this would let a sunbeam through, heating the optics. “It was one of those arcane little details that you’d never guess until you start getting into it and you start finding out, ah, Christ, five of the pinholes are lining right up and that’s going to let sunlight right in,” Menzel said. “It doesn’t sound like much, but it was driving Andy to drink. And God bless him, he figured it out.” Tao diligently sought out a suitable configuration of pins so that the holes in the five slightly-different-size Kapton layers would never align from any angle.
The moles were being whacked so slowly that astronomers began referring to the situation as “the JWST problem.” Back in 1996, Mather and his team estimated that the telescope would cost $564 million — a somewhat disingenuous guess aimed at getting Congress on board — and that it would launch in 2007. As the price tag soared and the launch date hopped ever farther into the future, Congress grew impatient. In 2011, JWST was nearly canceled, but elementary school students wrote letters to Washington and Senator Mikulski again came to NASA’s rescue.
Glass, metal and plastic gradually got bolted together in clean rooms at Goddard, Northrop Grumman, Ball Aerospace and elsewhere. But the assembled hardware couldn’t simply be sent skyward, because the telescope is going a million miles away, where astronauts with wrenches can’t visit. As Northrop Grumman engineer Jon Arenberg once put it, “This is a one-strike-and-you’re-out business.” Webb must deploy flawlessly on the first and only try. That means it had to be extensively, painstakingly tested on the ground. And in 2017 and 2018, these tests turned up one problem after another.
After a “shake test,” a bunch of screws and washers that had held the sunshield cover in place were found on the floor; they hadn’t been properly torqued. Another time, the sunshield caught on a snag and tore. Once, it unfolded, but not without a string wrapping around something it shouldn’t have.
The telescope got in another scrape when it was shipped to the Johnson Space Center in Houston, placed in the chamber where Apollo astronauts once practiced their moonwalks, and cryogenically cooled to simulate the conditions of outer space. While instrument builders like Sarah Kendrew were testing the cold hardware, Hurricane Harvey hit. The whole city catastrophically flooded, but the biggest worry for the Webb team was the liquid nitrogen supply. If it ran out, the telescope’s temperature would rise too quickly, damaging the instruments. The liquid nitrogen suppliers had to be urged to send trucks through the floodwaters as a matter of national importance.
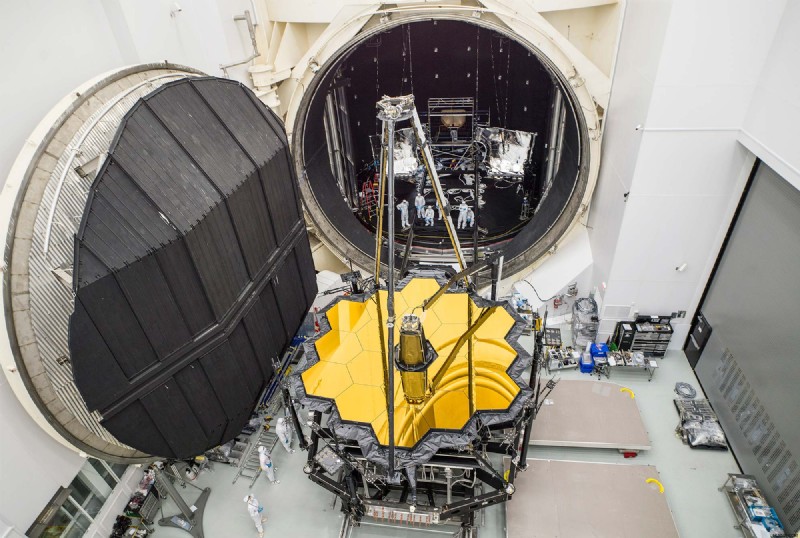
Problems continued. Earlier this year, the transponders that will blip data back to Earth were found to be faulty and had to be repaired. “A delay causes its own cascade of issues,” Tremblay said — and more expense:“It costs $10 million a month just to keep James Webb on the clean room floor.” As the investment rose, so did the need for the mission to succeed. “If NASA was willing to accept more risk, JWST would have been half the cost,” Tremblay explained.
Finally, problems abated. Northrop Grumman engineers successfully unfolded the sunshield several times at its facility in Redondo Beach, California. But according to Menzel, even after the shimmery layers have spread smoothly open, “we aren’t as elated as you might think. Because we all know that sunshield will only be as good as the last time it’s folded.”
Webb’s final cost approaches $10 billion. That’s nearly 20 times its sticker price, but still a few billion less than an aircraft carrier. Following some final pandemic-related delays, late 2021 became its target launch date. In September, the telescope passed a final test in Redondo Beach:Gold ears pinned back and blanket stashed away, the whole observatory was turned on its side, then righted, then checked to see whether anything had changed. Then it was put back on its side into a shipping container and taken away. Garth Illingworth, who has been involved with the telescope in a range of capacities since the beginning, went to Redondo Beach to see it off. There it had stood, “tall and majestic,” he wrote in an email. “By the next day the clean room was even empty of any JWST-related support hardware.” The exact date of the shipping container’s departure from California was kept quiet — a precaution against piracy on the high seas — but in early October it voyaged through the Panama Canal to French Guiana, a region near the equator where the European Space Agency launches its plus-size Ariane 5 rocket to exploit the extra kick of Earth’s rotation.

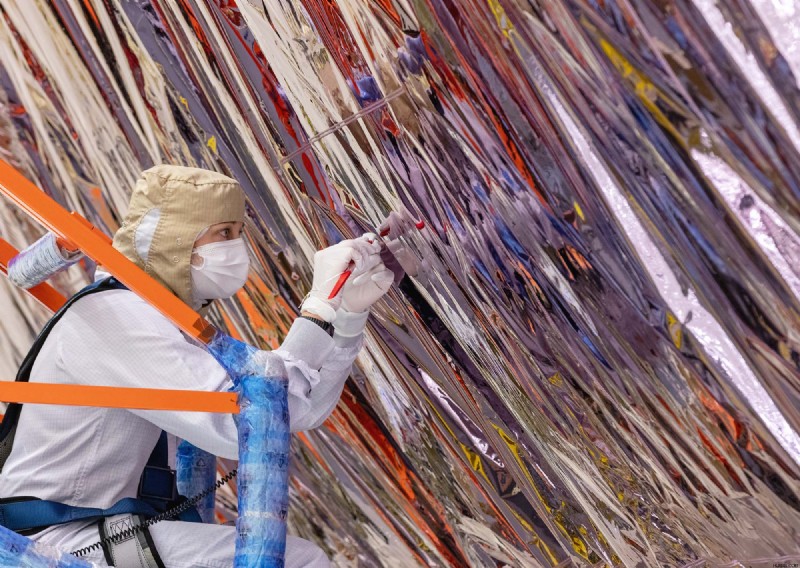
The Webb team is busy rehearsing the routines they’ll execute 24/7 out of Baltimore during Webb’s monthlong journey to Lagrange point 2, followed by its five-month commissioning period. “Do I feel confident?” said Menzel. "はい。 I feel confident that we’ve done everything we possibly could. The risk is acceptably low. It’s as good as it’s going to be. And I’m pretty confident that we’re going to do fine.
“Could something go wrong? Hell, yeah.”
Reasonable Guesses
Once the Hubble got working, humanity soaked up the sight of the cosmos like near-sighted kids wearing glasses for the first time. We also learned there was stuff out there that we couldn’t see.
In 1998, two rival teams of astronomers used the Hubble along with other telescopes to observe supernovas in distant galaxies and ascertain that the expansion of the universe is accelerating. This exposed the existence of an accelerating agent infusing all of space, known as dark energy. There’s so much space that dark energy makes up 70% of everything. (Another 26% is dark matter, and 4% is luminous atoms and radiation.)
Other puzzles soon turned up. The astronomer Wendy Freedman used Hubble to observe pulsating stars called cepheids. From these, in 2001 she and her team measured how fast the universe is currently expanding, achieving 10% accuracy, a huge improvement over previous measurements. In the years since Freedman’s measurement, the cosmic expansion rate has landed at the center of the biggest controversy in cosmology. The issue is that, based on the universe’s known ingredients and governing equations, theorists infer that space should currently be expanding more slowly than the measurements suggest. Its fast expansion may point to additional unknown ingredients in the cosmos beyond dark matter and dark energy. But Freedman, who is calm and authoritative, isn’t convinced yet that the measurements are right. She’ll lead a team that will use the Webb telescope to scrutinize cepheids and other stars more closely; they hope to measure the expansion rate precisely enough to tell for sure whether there’s an exotic fundamental ingredient afoot.
Meanwhile, the Hubble Deep Field photo told a rollicking story of galaxy evolution that dramatically expanded human knowledge of cosmic history. But it remains to Webb to read the crucial first couple of chapters of the story.
Marcia Rieke, a longtime professor at the University of Arizona regarded as one of the pioneers of infrared astronomy, has spent the last 20 years overseeing the design and construction of NIRCam (as in “near-infrared camera”), one of Webb’s four main instruments. She and her team at Arizona are planning to use more than half of their whopping 900 hours of guaranteed telescope time to do a new deep-field survey, one that will peer deeper into the past than ever before. Whereas Hubble could see the faint smudges of galaxies at redshift 10, corresponding to 500 million years after the Big Bang, Webb should be able to see those smudges very clearly and spot brand-new galaxies germinating farther away, perhaps as far back as 50 or 100 million years after the Big Bang.
Rieke and her team will do one better than the Hubble deep field. After using NIRCam to get an image of their dark patch of the sky, they’ll identify the galaxies in the patch that are farthest away and use NIRSpec, Webb’s near-infrared spectrograph, to take the galaxies’ spectra, from which Rieke and her colleagues can deduce their chemical compositions.
The spectrum will show which elements of the periodic table existed in each proto-galaxy, and how their elements evolved over time. The standard story is that early gas clouds, stars and galaxies mostly consisted of hydrogen, and supernovas and other explosive events gradually forged heavier elements. “But there are curious things,” Rieke said. “Close to the limit that Hubble can go to, there are quasars” — super-bright centers of galaxies powered by supermassive black holes — “and it looks like they have almost the same elements as the sun. Which is hard to believe. So there’s something that goes on early that we don’t have a good handle on.”
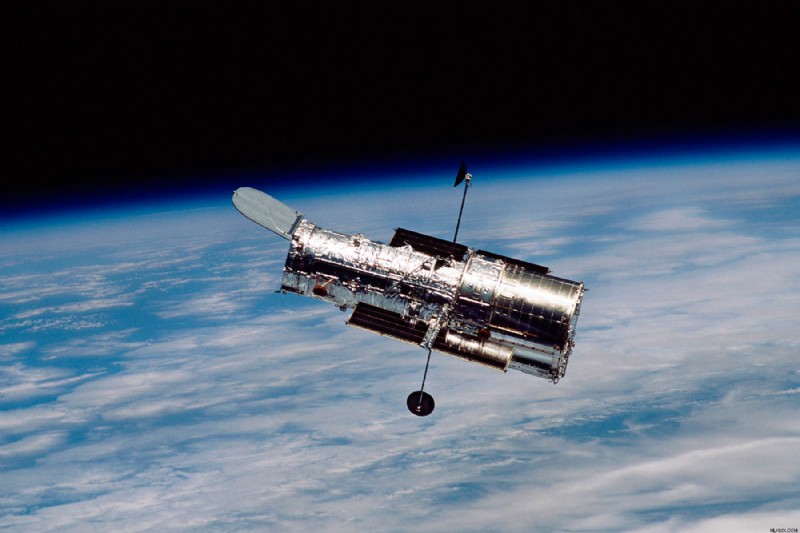
There are as many reasons for wanting to see the first stars and galaxies as there are astronomers, astrophysicists and cosmologists. For Risa Wechsler, a cosmologist at Stanford University, it’s a way to watch dark matter’s handiwork. She and her colleagues will use the proto-galaxies to deduce the distribution of sizes of dark matter halos that must have existed in the early universe, and when they formed. This can reveal whether dark matter is “cold,” that is, made of slow-moving particles, or “warm,” since particles that whizz around would have taken longer to huddle into halos. This temperature check would be a significant clue to dark matter’s nature.
Other researchers want to understand the first stars. Some think Webb will see so-called “Population III stars,” primordial beasts that are hypothesized to have been roughly 10,000 times heavier than our sun. Such stars would help solve another major mystery of galaxy formation:how galaxies’ centers ended up with supermassive black holes — physically small yet incredibly powerful gravitational sinkholes that can weigh billions of times the mass of our sun. Nobody knows how supermassive black holes grew so heavy, or when, or why their properties are correlated with properties of their host galaxies. One theory is that Population III stars seeded the holes, but there are a million other theories. Webb will look for signatures of the different scenarios.
Theorists have simulated many possibilities for how structures might have emerged in the young universe. But they can’t simply start with the cosmic microwave background and evolve that picture forward on the computer to see what happened. “A lot of the initial conditions are not well understood — things like the magnetic field, and how much turbulence there is in the gas,” said Peter Behroozi, a theorist colleague of Rieke’s at Arizona who simulates star and galaxy formation. It’s “a lot of work,” he said, to get from a large, ever-so-slightly dense spot in the cosmic microwave background to a tiny cloud of gas that will gravitationally contract and make a star.
“Oftentimes what people will do is, they’ll just skip that,” Behroozi said, and “start with a spherical cloud of gas. They don’t know what the distribution of [dark matter] clump sizes will be, so they guess. They don’t know about the magnetic field; they don’t know anything about the spin or turbulence of the gas, so they’ll fill all that in with guesses.”
The guesswork has accelerated recently as researchers race to get their predictions on record before Webb shows how star and galaxy formation really went down. Even conservative guesses can produce simulations with wildly different outcomes. “The main conclusion from my research,” Behroozi said, “is even if you try to make a reasonable guess, we still have no clue what James Webb will see.”
Bridge Planets
Natasha Batalha, Natalie Batalha’s second child, was 18 when she went to Florida for the Kepler launch. She remembers tagging along with her younger sister in the viewing room where the NASA science team had gathered. “During the launch, the anxiety that was in that space was chilling,” she said on a recent video call. After liftoff, the scene turned jubilant. She found it inspiring to see a team accomplish something so grand, but it was the possibility of discoveries being so close at hand “that was really what flipped on my brain to start thinking about exoplanets as a concept,” said Natasha Batalha, who is serious and precise, like her mother.
Space wasn’t a constant topic of discussion in the Batalha household. “I didn’t want to saturate their lives with science,” Natalie Batalha said. “I always wanted them to feel like they were number one.” But she and Celso did have “a secret hope” about Natasha. One evening in 1996 while Celso was teaching a night class, Natalie loaded up the half-asleep kids to drive them to a meadow so they look for the passing comet Hyakutake. Before they’d pulled out of the driveway, five-year-old Natasha said from the back seat:“What’s that?” She was pointing at the comet.
When Natasha was eight and living in Brazil, her mother asked her and her siblings to draw an astronomer. Natasha drew a white man, and Natalie asked her why. “This was crazy for me, the daughter of a Latinx scientist and a female scientist; I still had these stereotypes ingrained in my mind,” Natasha said. She suddenly felt empowered by the thought that she could belong in science.
A couple of years later she read Sally Ride’s autobiography. Just as her mother had been inspired by Ride, Natasha decided to become either an astronomer or an astronaut. She dreamed of being the first person on Mars. After the Kepler launch, as more and more exoplanets were discovered, she grew interested in the possibility of extraterrestrial life and how we might infer its presence on those planets through telescope observations of their skies. She got a dual Ph.D. in astronomy and astrobiology. Then, soon after Kepler ended and her mom left NASA Ames to become a professor at Santa Cruz, Natasha took a job at Ames studying exoplanet atmospheres.
Natasha Batalha is part of a growing research community whose ultimate goal is to detect “biosignature gases” — gases in a planet’s atmosphere that could only be there because of life. Every kind of molecule has characteristic wavelengths that it absorbs. So by collecting light from a star when a planet is and isn’t transiting in front of it, and checking which wavelengths of starlight grow dimmer when the planet is there, you can see which molecules are present in the planet’s sky.
Oxygen is an obvious candidate for a biosignature gas:It’s so reactive that it’s unlikely to be found unless the planet’s oxygen supply is continually replenished by, say, a biosphere doing photosynthesis. Photosynthesis is such a simple and efficient energy-capturing procedure that astrobiologists think it’s likely to evolve on any living planet, so oxygen is smart to look for.

But seeing oxygen by itself wouldn’t necessarily be convincing. Computer simulations show that under some conditions, oxygen can fill the skies of lifeless planets. “The challenge is there’s not one gas that’s going to be a biosignature gas,” said Hammel, the planetary scientist. “Methane on Earth is mostly produced by cows … but you look at Neptune, and you can see tons of methane there, and that’s not produced by cows.”
A better biosignature is a peculiar mix of gases. “It’s not going to be a single gas, it’s going to have to be a combination of gases in a configuration that tells us they are in a disequilibrium state,” said Hammel. “They can’t have formed that way naturally.”
Existing telescopes have already spotted molecular fingerprints in the skies of hot Jupiters, but these are lifeless planets. Detecting the weaker signals from rocky, possibly habitable planets’ skies will require JWST. Not only will the telescope have close to 100 times Hubble’s resolution, but it will see exoplanets far more clearly against the background of their host stars, since planets emit more infrared than optical light, while stars emit less. Importantly, Webb’s view of exoplanets won’t be obscured by clouds, which often prevent optical telescopes from seeing the densest, low-altitude layers of atmosphere. “Imagine being in a plane and looking down at an insane cloud deck, and you can’t see the surface at all,” Natasha Batalha said. “When you look at infrared light, you can all of a sudden see through the cloud deck.”

Exoplanets will be among the targets in JWST’s “Cycle 1” round of observations, which it will start tackling as soon as deployment and commissioning are complete — about six months after launch. The exoplanet community elected Natalie Batalha to lead transit spectroscopy studies of three gas giants as part of these early observations. Her team will also develop data pipelines and processing techniques for the community to copy.
Cycle 1 also includes observations for specific groups of astronomers. Last year, more than 2,000 groups submitted proposals to use JWST in the first cycle; a time allocation committee selected 266. Dozens of these programs will look at planets. When I video-chatted with Hammel, she screen-shared PowerPoint slides highlighting various exo-worlds of interest that Webb will turn its eye toward on behalf of different observers:Kepler 16b, which orbits two stars; the suspected “lava world” 55 Cancri e; and the seven rocky planets of the nearby Trappist-1 star system. (Hammel, who gets 100 hours of guaranteed observer time as a longtime member of the Webb science team, will browse our own solar system, including Jupiter’s red spot, the mysterious, far-flung objects of the Kuiper belt, and Hammel’s oft-overlooked favorites, Uranus and Neptune, which appeared as a pair of plush toys behind her on her office couch.)
Out of all the exoplanets that JWST will look at in Cycle 1, Natasha Batalha reckons that the three Trappist planets that orbit in their star’s habitable zone probably have the best shot at featuring detectable biosignature gases. “The Trappist system is unique in that the star is very small, and so the relative feature size of the atmospheres doesn’t need to be big in order for you to be able to see it,” she said. Whether Webb has a realistic chance of spotting biosignature gases is debatable, however. “Often the controversy comes up over the detection of oxygen,” she said. Oxygen absorbs one infrared wavelength in Webb’s range of sensitivity, and so theoretically an oxygen-rich transiting planet could put a noticeable dip in its star’s spectrum at that wavelength. “However,” she said, the wavelength “is just at the edge where the detector loses sensitivity.” Other types and combinations of gases will be easier to detect but might be harder to definitively attribute to life.
Webb might just identify possibly living planets, which would then be examined more closely with future space telescopes. Astronomers are busy planning those now. NASA’s Nancy Grace Roman Space Telescope, slated to launch later this decade, is mostly designed to study dark energy; Earth-like exoplanets are the purview of a future telescope concept provisionally known as LuvEx, an ultraviolet, optical and IR telescope that (if funded by Congress) will launch in the mid-2040s.
What we will look at then depends on what we learn in the next few years.
One morning this spring, Natasha Batalha woke up to a text from fellow exoplanet astronomer Johanna Teske:“We got it!” The 266 selected Cycle 1 programs had just been announced, and a proposal led by Batalha, with Teske as her deputy, made the list.
Theirs will be the most extensive of all the exoplanet observing campaigns in the first cycle:a 142-hour survey of super-Earths and sub-Neptunes, the ubiquitous midsize “bridge” planets that our solar system lacks, and whose composition, habitability and formation history are unknown. Assuming that, over the next few months, everything unfolds as it should and the James Webb Space Telescope finds its focus, it will point at 11 of these planets on behalf of Natasha Batalha and her team. When she awoke to the good news, first she called Teske. Then she called her mom.
Corrections: June 3, 2022
The original version of this article featured an image of the M100 galaxy that was incorrectly identified as having been taken by the Hubble Space Telescope. That image has been replaced with the correct Hubble image. In addition, the original article also incorrectly stated that Hubble does not have a spectrograph. Hubble now has two spectrographs; both were added to the telescope after the Hubble Deep Field image was taken.